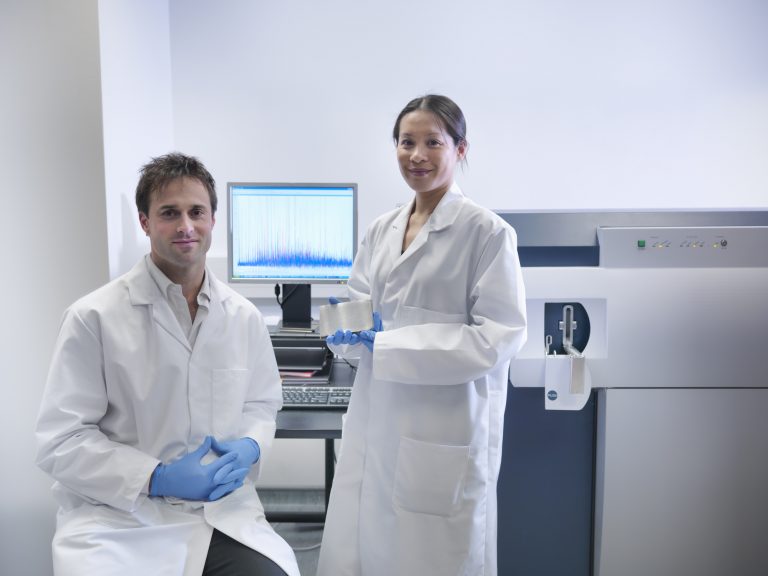
The possibility of metabolomics playing a role in cancer diagnostics and treat- ment seems, to many metabolomicists, a question of when rather than if. After all, metabolomics is a powerful platform to measure the endpoint of human physiology—a direct readout of physiological changes—and is easily sampled in blood. Despite its usefulness, there are multiple hurdles on its path to clinical appli- cation for oncology. But, as more people realize the doors that metabolomics can open for cancer research and treatment applications, getting over those hurdles seems more manageable than ever.
Metabolomics is loosely (and insufficiently) defined as the study of metabolism. However, Shankar Subramaniam Ph.D., chair and professor of bioengineering at University of California, San Diego School of Medicine, told Clinical OMICs that there are many, expansive questions in the field. Some researchers are interested in identifying markers in the blood that are indicative of various tumors—using metabolites as yardsticks for cancer. For example, the increase of proline, threonine, aspartic acid, betaine, and dimethyl glycine in serum of patients with colorectal tumors. Or, the increase of linoleic acid and choline in lung cancer. Caveats abound, however, as many markers are late markers and they are more correlative than causal.
A mechanistic origin of altered metabolism in tumors is also an area of interest. Most tumors are in a hypoxic environment and the cells need to find alternate sources of non-oxygen driven metabolism. If the mechanistic origins of altered metabolism in cancer can be understood, alleviating the growth and progression of tumors as well as discovering therapeutic strategies could be possible. Also, drug metabolism could lead to improvements in the metabolism of that particular drug and combinatorial therapy.
In order to understand metabolomics in cancer, Subrama- niam explained, we have to start at the Warberg effect. The Warberg effect describes that cancer cells favor glycolysis rather than the oxidative phosphorylation—the ATP production method utilized by the other cells in the body. This discovery, described in 1927 in the Journal of General Physiology for which Otto Warberg was awarded the Nobel Prize in 1931, has been a topic of discussion for decades, according to Subramamiam. And, the research surrounding this metabolic phenomenon has increased in the last twenty years as people have started to question the altered metabolism illustrated by cancer cells. Now, almost a century later, the field is moving with break- neck speed. Oliver Fiehn, Ph.D., professor at the University of California, Davis, and director of the
West Coast Metabolomics Center, noted that “it is great that metabolism is recognized as a hallmark of cancer and that researchers and clinicians embrace this idea from finding cures to other interventions.”
The poster child
The promise held by metabolomics to advance cancer treat- ment is perhaps best illustrated by the seminal 2009 Nature paper illustrating a causal association between genetics, metabolism, and cancer. The collaborative paper, “Cancer-associated IDH1 mutations produce 2-hydroxygluta- rate” showed that mutations in the isocitrate dehydrogenase 1 (IDH1) gene lead to production of an oncometabolite, 2-hydroxyglutarate (2HG). The excessive 2HG accumulates, contributing to the formation and malignant progression of gliomas. Many of the authors on the landmark paper were from Agios Pharmaceuticals, a company that has turned this metabolomic discovery into a druggable target.
With two drugs having been granted FDA approval, TIBSOVO (Ivosidenib) and IDHIFA (Enasidenib), Agios is successfully bringing metabolomics and cancer from the bench to bedside. IDHIFA was FDA approved in August, 2017 for patients with relapsed or refractory acute myeloid leukemia (AML) who have IDH2 mutations and TIBSOVO was granted FDA approval in July 2018 for patients with relapsed or refractory acute myeloid leukemia with an IDH1 genetic mutation.
Metabolomics was the key to finding how the genomic alteration changed the cancer, noted Andreas Huhmer, Ph.D., senior director, proteomics and metabolomics at Thermo Fisher Scientific. He adds that because metabolomics is “very actionable” it allows the study of how organisms react to change which is more difficult to study using only genomics.
Building the foundation
A disease state recognized by deviations from the healthy state can only be recognized when the normal profile is established. “It is only then that deviations become mean- ingful,” noted Subramaniam. Therefore, baselines must be established—perhaps the creation of a Human Reference Metabolome, of sorts.
But, it takes a lot more to organize a metabolomic database than a genomic one. “There are 23,000 genes, but there could be millions of metabolites” noted Teresa Fan, Ph.D., professor at the University of Kentucky Markey Cancer Center. Although a reference metabalome may not be possible due to its vast complexity, building large databases of metabolomes is work that is at the heart of metabolomics today. Nightingale Health in Helsinki, Finland and UK Biobank announced plans in the summer of 2018 to analyze metabolic biomarkers in 500,000 blood samples. The work, which is funded by Nightingale and uses its biomarker profiling technology, will be incorporated into UK Biobank’s public database— after a nine-month period during which Nightingale has exclusive access.
Subramaniam heads up an NIH-funded project known as the Metabolomics Workbench—creating a public, interactive repository for metabolomics metadata and experimental data “spanning various species and experimental platforms, metabolite standards, metabolite structures, protocols, tutorials, and training material and other educational resources.” Just last fall, the University of California San Diego received a $12-million, four-year grant from the National Institutes of Health to expand the workbench.
Other databases are being built, including the Genome Canada-funded Human Metabolome Database (HMD- B)—a comprehensive, openly accessible, online database of human small molecule metabolites—created by the Human Metabolome Project. In addition, the Biological Magnetic Resonance Data Bank (BMRD) collects, annotates, archives, and disseminates spectral and quantitative data derived from NMR spectroscopic investigations of biological macromolecules and metabolites.
Although the focus of these large resources is not can- cer, specifically, the growth of information will undoubtedly have far reaching effects on the field as a whole. And, because metabolomics reaches deep into the nooks and crannies of all human physiology, gains in cancer metabolomics are sure to follow.
“Contract research organizations (CROs) that conduct metabolomics (such as Metabolon) have improved over the past decade” said Chen Dai, Ph.D., a recently defended graduate student in Laurie Littlepage’s lab at University of Notre Dame. Now, continued Dai, “we have a much more complete understanding of metabolism as a system, and much better databases with the spectra of thousands of metabolites, which allows for identification of more metabolites than ever before. Such advances in the tools has brought great discoveries, one of which is the discovery of oncometabolites.”
It is not only the detection of metabolites that is important, but the tools that allow for the analysis of huge datasets which should facilitate the systematic study of metabolism. “Metabolism is notoriously complicated, and that complexity creates problems in research as altering one component can have unforeseen effects across many other pathways, which is really difficult to analyze” noted Dai. He adds that new tools “allow for the integration of metabolomics data with proteomics and transcriptomics data, presenting a systematic picture of the interactions and changes.”
In addition, Andrew Lane, Ph.D., professor at the Uni- versity of Kentucky College of Medicine, asserts that using the metabolomics approach for the purpose of diagnosis, but also prognosis and monitoring response to intervention, “relies very heavily on statistical analysis.” He added that effect sizes are frequently quite small when looking for a few metabolites out of thousands in blood, which has been in contact with every part of the organism. Although numerous claims for high-accuracy biomarkers have been made, noted Lane, to date none of them have been shown to be robust.
“Engaging scientists from multiple disciplines, including from biostatistics, computational science, analytical chemistry, and engineering, is leading to significant achievements in developing new tools that can be used to address challenges within the field,” added Laurie Littlepage, Ph.D., assistant professor of cancer research, University of Notre Dame.
In order to answer the big research questions, associating a measurement with a biochemical pathway or a fundamental mechanistic function relies on deciphering and understanding the complexity of the metabolic pathways of human physiology. “We need to try to get a metabolic map,” noted Subramaniam. This is the big challenge for the coming decade—to understand the complete human metabolome. But, he added, that the pieces are starting to fall into place.
Going where genomics cannot
Targeting events that are an “Achilles heel” for cancer cells is how the University of Kentucky’s Fan is pioneering metabolomics in cancer. Using 3D cultures from patient-de- rived organoids, Fan tests how individual patients respond to different drugs. Using 96-well plates, the Fan lab grows the organoids to which a tracer can be added to track the metabolism in the presence of a drug. Upon quenching the metabolism, they then measure the cell’s response to the drug metabolically using both NMR and MS. In the end, they are trying to understand the mechanism of how the drug impacts the cancer cell through metabolism.
Fan is pioneering research that will someday help patients receiving immunotherapeutics. Fan said that one of the powerful aspects to using metabolomics is harnessed with the plasticity of the immune system in patients. This is especially true with immunotherapy, where the efficacy can be dictated by the tumor microenvironment. For example, if the macrophage prevents the T cell from getting to the tumor, the therapy will not be efficacious, leading to poor patient survival.
Genetics cannot predict these findings, Fan said. Genomic screens are useful if the mutation has to do with the drug effects, but she finds that is not often the case as there are many examples where the environment determines the efficiency. Fan noted that we “need to put all of the omics together to tease apart a patient’s complexity.”
Some researchers work to map out metabolic landscapes of specific cancers. For example, recently published work in Cancer and Metabolism from the Littlepage Lab identified specific metabolic changes occurring during breast cancer, not only as com- pared to normal tissue but also as induced by multiple
individual oncogenes. The team mapped out the metabolic landscapes of a few commonly used genetically engineered mouse models of breast cancer, a useful resource for peo- ple who use these models to mimic human breast cancer patients to understand the effect of oncogenes. “Learning how individual molecular alterations perturb the metabolic landscape is essential to our understanding of how altered metabolism can be utilized as a vulnerability and targeted with therapy,” said Littlepage.
The long and winding road
The databases currently being built still are not broadly applicable in the clinic. In order to use metabolomics for diagnostics, and identifying a specific biomarker which cannot be detected with other, cheaper tools is necessary. Not only that, metabolomics is expensive and analysis can be time-consuming. So, a facility capable of quickly and cost-efficiently detecting the biomarker is also required.
One researcher noted that it cost “over $20,000 for 36 sam- ples to run and analyze, and it took months to get the final data analyzed.” Even after all of this, due to the various different sample preparation methods and separation techniques, there might be metabolites whose levels are biased and may need to be verified with other methods. Even if all of the data is absolutely accurate, there are still not nearly as many good metabolic biomarkers as there are genetic ones. Sample collection from patients is another important consideration of bringing metabolomics to the clinic.
Despite the challenges that surround the sheer enormity of building a metabolomics database and other aspects of metabolomics, the researchers in the field see their work playing a role in advancing cancer treatment and diagnosis in the future. As Fiehn noted, it is “a long way to go from a cool idea to use as a standard in care.” But, metabolomics is uniquely positioned as a readout of mechanism and func- tion. It can take many different studies to ascertain what a genetic mutation does and generations to see changes. However, in metabolomics, a change can be measured in minutes. With its many advantages and challenges, Fan said that “metabolomics is at that early stage—just like where genomics and proteomics started. But, we’ll get there.”