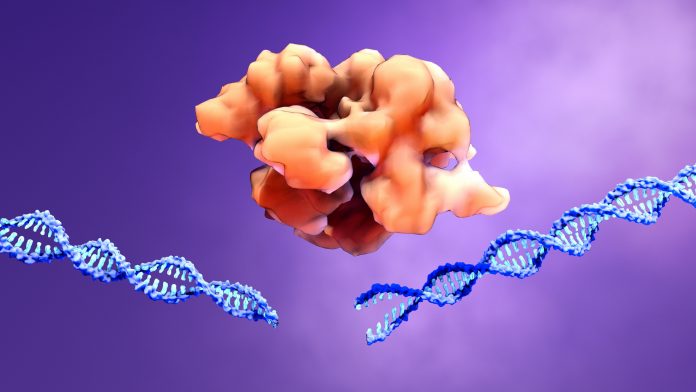
Researchers at the Massachusetts Institute of Technology (MIT) report that their new approach of using CRISPR-based prime editing provides a faster and more efficient way to screen for cancer mutations in individual genes in their natural setting. This will allow researchers to better understand the role those mutations play in the development, progression, and treatment response in a tumor.
Their work, published today in Nature Biotechnology, focused on the tumor suppressor gene p53, shows that editing the genome as opposed to introducing an artificial version of the mutant gene is easier and faster than existing approaches—while also revealing that some p53 mutations are more harmful than they are currently considered. While this research focused only on screening cells with about 1,000 known mutations in p53, they note their new approach could be applied to other known oncogenes and could eventually be used to develop new precision approaches for treatment, based on predicting how an particular patient’s tumor will respond.
“In one experiment, you can generate thousands of genotypes that are seen in cancer patients, and immediately test whether one or more of those genotypes are sensitive or resistant to any type of therapy that you’re interested in using,” said senior author Francisco Sánchez-Rivera, PhD, an assistant professor of biology at MIT.
Sanchez-Rivera has been working with CRISPR genome editing technology for the past 10 years, beginning with using it to introduce lung-cancer linked genetic mutations into mouse models of the disease. Sánchez-Rivera and collaborator Tyler Jacks, PhD, at the time a post-doc and now a professor of biology at MIT, discovered they could delete genes that are often lost in lung cancer and the resulting tumors were similar to those tumors that naturally arise with those same mutations. At the time, the team was not able to create point mutations or insertions.
But a couple of years later, work by David Liu, PhD, of Harvard University and the Broad Institute, introduced the new development of base editing which allowed for the engineering of some, but not all, point mutations. Then, in 2019, Liu developed the prime editing technique, which allows for the insertion of any point mutation, as well as insertions and deletions. Liu is also an author of the current study.
“Prime editing in theory solves one of the major challenges with earlier forms of CRISPR-based editing, which is that it allows you to engineer virtually any type of mutation,” Sánchez-Rivera noted.
When commencing this current project, Sánchez-Rivera calculated that successfully executed prime editing could generate more than 99% of all small mutations seen in cancer patients. But this rate was dependent on the team finding a way to improve the editing efficiency and accuracy of CRISPR-based gene editing systems.
In this case, the prime editing guide RNAs (pegRNAs) are known to have varying levels of efficiency in cutting particular spots in the genome. This leads to “noise” in the data from pegRNAs that aren’t generating the right target mutation. The researchers solved this problem via the use of synthetic target sites that helped them devise methods to see how efficiently each pegRNA was working.
“We can design multiple prime-editing guide RNAs with different design properties, and then we get an empirical measurement of how efficient each of those pegRNAs is. It tells us what percentage of the time each pegRNA is actually introducing the correct edit,” said Samuel Gould, lead author of the paper and an MIT graduate student.
To show how this technique works, the investigators turned to p53 one of the most commonly mutated genes in cancer patients. From a dataset of more than 40,000 patients, they identified more than 1,000 different mutations that can occur in p53. Then, using their new method, the team introduced p53 mutations in human lung adenocarcinoma cells and measure the survival rates in order to find each mutation’s effect on the cells.
An important finding was that some of the p53 mutations promoted mor vigorous cell growth than previously thought from research that used a technique of inserting artificial copies of mutated p53 into a cell.
“This is a case where you could only observe these variant-induced phenotypes if you’re engineering the variants in their natural context and not with these more artificial systems,” Gould said. “This is just one example, but it speaks to a broader principle that we’re going to be able to access novel biology using these new genome-editing technologies.”
Currently, there are few approved drugs that target p53 since it is a tumor suppressor gene, which are difficult to reactivate. The MIT team, however, plans to study other mutations from cancer-linked genes in the hopes of informing the development of new therapies that can more effectively target these specific mutations. The hope is that eventually this new gene editing technique can be used to create new, personalized approaches to treating tumors with specific mutation profiles.