
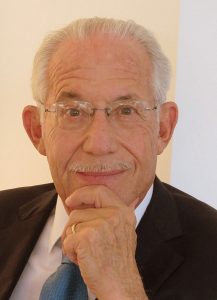
When I wrote about SARS-CoV-2 variants in the March edition of my column, no one could say with certainty how new variants of SARS-CoV-2 would change the course of the COVID-19 pandemic. But many public health officials and experts, myself included, feared they would cause serious problems. In the months since, this prediction has become our reality, casting a long shadow over our collective attempts to control the pandemic and prevent further death and disease. CDC Director Rochelle Walensky has gone on record to say that a vaccine-proof variant might be “only a few mutations away.”
Initially, variants from different geographical regions exhibited similar mutations, fueling speculation that the virus had only a limited number of changes within reach. Over time, the hope was, SARS-CoV-2 would assume an evolutionary trajectory similar to that of cold-causing coronaviruses, becoming no more harmful than another common cold. Though not far-fetched, this scenario was dubious for several reasons. One, this did not prove to be the case for a virus very similar to SARS-CoV-2, influenza, which requires new batches of flu shots each year to account for its rate of variation. Two, the behavior and virological profile of SARS-CoV-2 is demonstrably different from that of its more benign cousins.
What we didn’t know then—though a mounting pile of evidence has since confirmed—is that SARS-CoV-2 does, in fact, have a tremendous capacity for adaptation and change. We’re also beginning to understand why.
First and foremost we must consider the ecological nature of this virus. Every species has its niche—the place it occupies in a broader ecosystem. Viruses are no exception. Some viruses, like respiratory syncytial virus, affect mostly newborns and children. Others, like HIV, hepatitis B, and syphilis, are sexually transmitted and circulate among humans by way of our intimacies.
SARS-CoV-2 falls into a third category: influenza and cold-causing viruses. Their ecological niche is much broader, consisting of long-lived, immunocompetent, gregarious adults who have been previously infected by the selfsame virus. Viruses that occupy this niche develop the capacity to reinfect their human hosts over and over again, no matter the immune defenses we develop in response. Viruses possess nothing remotely like human intelligence, but they do have time on their side—a rapid mutational variation akin to artificial intelligence. Each generates countess mutations. The variants that allow them to continue to spread through a population come to dominate.
Remarkably, we were able to develop vaccines that protected against the original strain of SARS-CoV-2 in less than a year. But studies conducted in the wake of the emergence of the Alpha, Beta, Gamma and particularly Delta variants show that the strength and durability of that protection might not hold if the virus continues to become more virulent and, in the worst-case scenario, more lethal.
What follows is an overview of what we’ve learned over the course of several months of studying the variants.
Lesson 1. We don’t know how much the virus will evolve, but it is possible we’re only in the beginning stages.
Avidity
To initiate and sustain infection, SARS-CoV-2 binds to the ACE2 receptors that are expressed in abundance across our airways and nasal passages. The tighter the virus binds to the cells of the nasal mucosa, the fewer the number of particles required to infect—a definite selective advantage.
Just how avid could the virus become? According to a recent study the limit is far beyond what new variants have exhibited thus far. Scientists were able to increase its binding capabilities 600-fold. The avidity of SARS-CoV-2 variants currently in circulation is likely lower by several orders of magnitude.
Some changes in avidity seem to balance other changes in immune evasion that may render the mutant less fit. Further study of such interactions is needed to see whether they could play a role in vaccine and drug development.
Sites of mutation and potential drug targets
The primary target of the neutralizing antibodies in COVID-19 vaccines and monoclonal antibodies in certain drugs is the spike protein, which contains the receptor binding domain that affixes to ACE2. Several of the mutations identified across the new variants are located on the spike.
One region of the spike protein can be thought of as the primary antigen of the primary neutralizing enemy—a waving flag that lures antibodies its way. Remarkably, this part of the spike, called the receptor binding domain, can mutate to decrease immune recognition and still bind to the ACE2 receptor to permit virus entry. Sometimes, when the receptor binding domain changes to avoid immune detection, the mutations it incurs may actually decrease infectivity. We’re now learning that changes occurring outside the receptor binding domain can regain these losses through adjoining mechanisms. Called compensatory mutations, these changes ensure the virus can still evade immunization while simultaneously becoming more infectious.
Examining the full extent of variation across the viral genome, we see that across the vast spectrum of cold-causing coronaviruses, the protein sequence of the entire spike diverges only two to four percent every eight years, while divergence in the receptor binding domain, at its peak, reached 17 percent. The conclusion is, once again, that SARS-CoV-2 may be nowhere near the limits of its own variation. The latest round of variants including Mu, Lambda and C.1.2 show that twenty months into the pandemic SARS-CoV-2 is still developing new variants that may challenge our best vaccines.
Lesson 2. The virus selects for changes that increase its ability to jump from one person to another.
Stability of the virus particle
The survivability of SARS-CoV-2 is directly dependent upon its transmission from host to host. Mutations could enhance this ability by increasing the stability of the virus particle and how long it can maintain structural integrity outside the host environment. Some the new variants include mutations in the structural proteins E, M and N. These should be investigated not only for their effect on virus replication but also for the possibility that they confer increased stability to the free virus particle, a property which could provide a selective advantage.
Immune suppression
SARS-CoV-2 is a master at evading both the defenses of an infected cell and the entire immune system. It is a tale of stealth and disguise worthy of any great mystery novel. The primary story, perhaps paradoxically, is one of immune suppression, not hyper-activation, which is seen so strikingly in those who fall seriously ill. To establish infection all viruses must first defeat the body’s well-honed defenses. Early on, the virus acts at multiple levels to counteract innate immunity, the first line of defense against microbial invaders, allowing enough time for the virus to enter and exit before the full set of antiviral defenses can mobilize.
The original SARS-CoV-2 virus encoded many proteins in its genome that modify and suppress the immune system. New variants appear to improve upon these existing capabilities, allowing the virus to replicate to high concentrations without drawing the attention and ire of cellular immune sensors. They take particular aim at interferon and the genes and proteins induced by interferon.
Beyond the structural proteins I already touched on—the spike, membrane, envelope, and nucleocapsid—SARS-CoV-2 has nonstructural proteins (NSPs) and accessory genes, including open reading frames (Orfs), that contribute collectively to this effort. Like Swiss army knives, the viral proteins of SARS-CoV-2 are multifunctional, with many performing several highly specific tasks. NSP3 alone encodes seven distinct functional domains, including protease cleavage, de-ubiquitination, de-ADP-ribosylation, and autophagy modulation.
All the virus’ mechanisms of immune suppression ultimately come in one of two forms: nonspecific and specific.
Nonspecific immune suppression
Nonspecific suppression, such as the obstruction of protein synthesis and export, indiscriminately affects entire cellular processes, even the machinery that potentiates viral propagation. This blunt apparatus of suppression forms an intensive blockade against immune signaling pathways, activated in coordination with counterbalancing elements that allow SARS-CoV-2 to continue replicating even as general cellular functioning is disrupted.
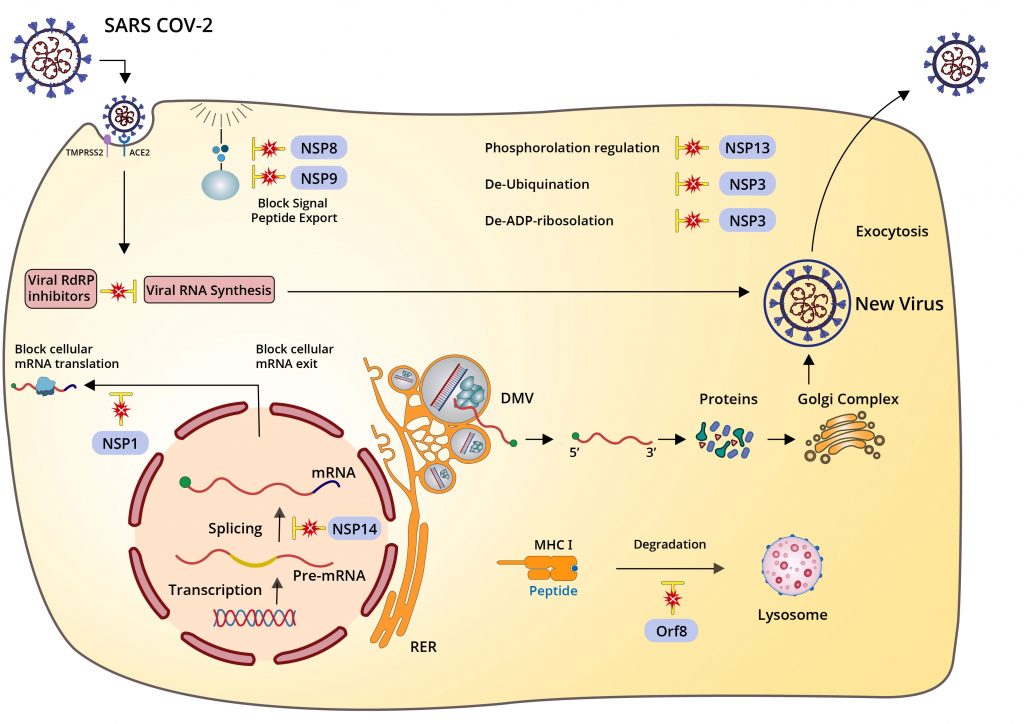
Source: ACCESS Health International
Specific immune suppression
Specific suppression is more precise as a mode of attack, zeroing in on microscopic structures, interactions, and processes with equally specific effects. Many of the Orfs, as far as we know, only interact with select innate immune signals, like interferon and interferon-responsive genes, while NSP1, in addition to its nonspecific functions, also specifically inhibits RIG-I, a pattern recognition receptor critical to the activation of the innate immune response.
Whether specific or nonspecific, any one of these mechanisms can, with the right mutations in place, increase in effectivity, which in turn will allow the virus to replicate more prolifically and reach higher titers. We now have evidence that this is what happened with the Alpha variant. Studies that used deep sequencing techniques to investigate differences in protein transcription and expression between the original and Alpha strains found an 80-fold increase in the amount of Orf9b subgenomic RNA produced by the Alpha variant and a ten-fold increase in the messenger RNA corresponding to the Orf6 proteins. Both Orf9b and Orf6 are inhibitors of innate immunity.
While we don’t yet understand the evolutionary trajectory of the Delta variant in depth, there is reason to suspect that its immunosuppressive capabilities are heightened compared to previous, less infectious variants. Even single point mutations in various genes are enough to substantiate change, the consequences of which have affected the development of influenza vaccines and hepatitis C drugs.
Lesson 3. We need to reevaluate our timeline and approach to pandemic control in light of what we know about new SARS-CoV-2 variants.
Taking these new findings into consideration, what is the bottom line for the future of pandemic control?
A report recently released by the Scientific Advisory Group for Emergencies (SAGE), an independent body of experts that advises the UK government in times of crisis, outlined four potential scenarios that can help frame our expectations for the future, as well as corresponding action items. They are as follows:
Scenario One: A variant that causes severe disease in a greater proportion of the population than has occurred to date. For example, with similar morbidity/mortality to other zoonotic coronaviruses such as SARS-CoV (~10% case fatality) or MERS-CoV (~35% case fatality).
Scenario Two: A variant that evades current vaccines.
Scenario Three: Emergence of a drug resistant variant after antiviral strategies.
Scenario Four: SARS-CoV-2 follows an evolutionary trajectory with decreased virulence.
To gain a deeper understanding of the paths laid out before us, we must first examine how a variant emerges and becomes dominant in a given population. The evolutionary potential of a virus is comparable to the computational power of machine intelligence. It doesn’t evolve by choice or design, but by generating random combinations of mutations until one rises above the rest as superior. Viruses aren’t the sole catalysts of this process, however. Ultimately the behavior of humans, as the SAGE report points out, is what changes the behavior of a virus like SARS-CoV-2. Over the course of the pandemic we’ve abandoned our previous way of life and gone to great lengths to protect ourselves from harm. Only variants that overcome the myriad obstacles we put in their way—including masks, vaccines, drugs, and basic prevention measures like social distancing—stand a chance of surviving.
Three specific enhancements, none mutually exclusive and all already documented, can improve the survival prospects of current and future SARS-CoV-2 variants. I described them independently of one another in the sections above but will summarize the sum total of their effects here.
The first is an increase in the speed and volume of replication activity. If higher concentrations of virus are produced in and released through our nasal passages, it makes logical sense they might reach a larger number of hosts.
The second is an increase in stability. The more stable the virus particle, the longer it can retain structural integrity and remain infectious.
Last but not least is an increase in avidity. The latest crop of variants has mutated from the original strain to stick tighter and longer to the surfaces of our cells—a trend that has potential to continue if, as in lab experiments, the avidity of the virus has an upper limit 600-fold greater than that of the original strain.
Scenario Five: Increased immune suppression
There is a possibility that the Delta variant represents the worst SARS-CoV-2 has to offer. But according to the SAGE report, this version of events—in which virulence decreases from here on out—remains unlikely, at least in the short term. That we’re only at the beginning of the variant taxonomical alphabet goes to show how much variation might occur before the tail end of this trajectory is in sight. Adding to the uncertainty is the epidemiological data we have on brutal COVID-19 surges that overwhelmed cities in India and Brazil. Despite the fact that their populations already had extensive exposure to the virus previously—in the case of Manaus, Brazil, enough to push past the theoretical threshold of herd immunity—they still suffered from high rates of hospitalization and death. Given that the Gamma variant originated in Brazil and the Delta variant in India, it is only fair to speculate that conditions in both places were ripe for the emergence of a variant that existing antibodies couldn’t neutralize.
There will likely be more variants to watch out for in the coming months. Currently on our radar are the Lambda variant, MU, prevalent in South America, and C.1.2 from South Africa. Sewer sleuths report the discovery of multiple novel, yet functional mutations in genomes exacted from treatment plants. Some of these variants encode changes that elude neutralization by sera of both those vaccinated or naturally infected as well as from approved monoclonal antibody treatments.
The current generation of COVID-19 vaccines is certainly preventing a reiteration of these catastrophic events in countries that have access to them, but their initial rollout has only gone so far. Even in a country like the US, where half the nation is fully vaccinated and 64 percent have received at least one dose, there are enough cracks in our collective defenses for SARS-CoV-2 to experiment with new recipes for increased infectivity and, by a stroke of our bad luck, crack the code for increased virulence, too. The SAGE report scenario in which SARS-CoV-2 evolves to become deadlier, killing one in 10 like SARS-CoV or even one in three like MERS-CoV, cites point mutations and recombination events as possible catalysts for this transformation. Such phenomena can come down to a shift as small as a single amino acid. Unless we act now to patch up the holes it might emerge from, who’s to say it won’t happen tomorrow?
In addition to the four SAGE scenarios, I would like to propose a fifth. Since the primary means by which SARS-CoV-2 entrenches itself among humans appears to be immune evasion and suppression, I could anticipate a turn of events in which SARS-CoV-2 becomes not just more adept at evading vaccines and drugs, but the immune system writ large. In this scenario, the period of asymptomatic transmission and duration of infectivity would be longer; the amount of virus produced, higher; and our defenses against the virus, fundamentally indisposed to neutralize it. This includes, of course, vaccines.
Effects on vaccines
Four components predict the effectiveness of vaccine protection. The first is how well the vaccine works against the original strain. Second, how long protection lasts. Third, how well it protects against variants. And fourth, individual variation determined by age, preexisting conditions, and so on. Of these four variables, the one we can control least is the duration of protection. But if what we’ve learned since January is any indication, it might also be our greatest cause for concern. However capable we may be of designing new vaccines that combat viral variation, there is only so much they can do if they can’t withstand the test of time.
We now know two things about the duration of vaccine-mediated immune protection that before, we could only suspect. First, the antibody concentrations elicited by vaccines wane over time; and second, SARS-CoV-2 has become increasingly adept at evading them. We can’t predict with precision how thorough their capacity for immune evasion may become, but if the virus goes the way of influenza and cold viruses, it could be disastrous—for the elderly and other clinically vulnerable populations especially. Already studies show that for individuals over the age of 60, the effectiveness of the Pfizer vaccine against the Delta variant—the best we have, along with Moderna’s—drops to 16 percent after just 10 weeks. Vaccines that fail to protect high-risk groups simply won’t cut it. As long as one population is susceptible to disease and death, we all are.
Recent data shows a third dose of existing vaccines, or adding one or more doses to vaccines approved as a single dose, will provide additional protection as the titer of neutralizing antibodies against both the original virus and the variants tested exceeds that of the original vaccination. Unknown is how long the additional protection will last and how effective it will be against new and emerging variants.
To combat a virus so proficient at adaptation, we need to bulk up and shore up our containment methods accordingly. Only one viable option exists for long-term disease control: a strategy that combines longer-lasting and broadly protective vaccines with antiviral drugs, rigorous public health measures, and sustained cooperation between governments, scientists, drugmakers, and health agencies. I call the sum total of these many layers of defense multimodal therapy. It requires no shortage of effort, funds, and coordination, but such lengths constitute the bare minimum of what it takes to beat SARS-CoV-2 at its own evolutionary game.

Multimodal therapy
Four layers of defense in total make up multimodal therapy. The first layer consists of COVID-19 vaccines—not just the ones we presently have on hand, but second and third generations and booster shots that protect against the variants and possibly even coronaviruses more broadly.
Antiviral drugs and prophylactics—drugs that prevent infection rather than treat it—form the second layer. Current COVID-19 treatments are experimental, high-cost, and limited in use to intravenous administration in clinical settings. Developing orally ingestible drugs that come in pill form would be of tremendous help in long-term care centers, schools, businesses, and other hermetic environments where infection spreads and must be contained quickly.
The third ring of defense comes from public health measures that curb spread on the national and population level, including widespread testing, exhaustive contact tracing, mandatory isolation, tight border controls, and quarantines for new arrivals. Such policies have had demonstrable success in countries like Australia, China, New Zealand, Singapore, and Taiwan. While not every nation currently has the infrastructure necessary for comprehensive testing, tracing, and quarantine programs, they should all work to build them.
But the first three layers of protection are only as good as the fourth and outermost ring: international cooperation. The international community needs to band together and invest in global disease surveillance, sequencing, and data-sharing. With this collective hub of information, scientists, researchers, and pharmaceutical researchers the world over must also combine expertise to determine how vaccines and treatments must be modulated in the face of new variants. Such an infrastructure would increase pandemic preparedness for decades to come.
For nearly two years COVID-19 has lived among us, and the new variants promise it isn’t going anywhere anytime soon. If we take what we’ve learned and use it to develop and implement long-lasting countermeasures against this virus and all to come, we will emerge from the current pandemic weary and battle-scarred, but stronger, wiser, and more resilient than before. The virus will only continue to adapt. The question becomes whether we can, too.